We are working mainly on high pressure fluids and fluid mixtures, with the main goal being to relate their atomic structure to the properties and behaviour of the bulk, macroscopic system. To this end we employ a wide range of techniques, from quantitative imaging, Raman spectroscopy inside Diamond Anvil Cells and neutron diffraction, to classical and ab initio molecular dynamics. An overarching goal of our ongoing research is to blend coherently theoretical and experimental methods to gain a holistic and accurate picture of disordered systems, from the atomic level to the macroscopic level.
Our feats to date detailing how Density Functional Theory-based Molecular Dynamics can be coherently blended with real-space methods for diffraction data refinement (using Empirical Potential Software Refinement – EPSR, which is a Reverse Monte Carlo-derived procedure for solving the inverse problem in disordered systems) were featured in an article on the cover of the Journal of Chemical Physics.
High Pressure Fluid Mixtures
A particular focus of our research is on non-random high pressure fluid mixtures, that is mixtures where the components preferentially form bonds (such as H-bonds). Of particular interest to us is hydrophobicity and what are the exact physical ingredients to produce or inhibit it.
During my PhD studies under the guidance of Dr. John Loveday we discovered that when moderate amounts of pressure are applied to fluid methane-water systems these begin to form homogeneous mixtures. This was an entirely unforeseen change in behaviour since methane is the textbook example of an apolar, hydrophobic ‘oil’-like molecule that is immiscible with water.
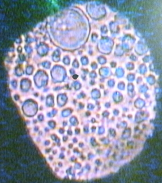
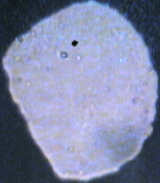
————————->
Increasing Pressure
Our attempts to model the behaviour classically failed completely to detect any effect of pressure on mixing, highlighting a fundamental inadequacy in widely-used currently available computer models, such as OPLS-AA for methane and SPC/E, TIP3P and TIP4P (rigid and flexible) for water.
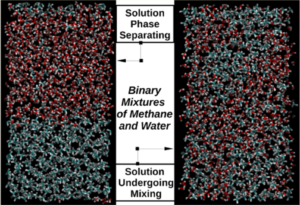
Subsequent investigations combining the first high pressure neutron diffraction measurement of a binary fluid mixture and very long first-principles quantum mechanical calculations seeded from the experimentally-extracted 3D structures of the fluid revealed this behaviour is associated with an unexpected dipole moment being acquired by the previously-believed apolar methane molecule.
This situation raises the question as to whether pressure-induced solubility is a property of methane alone or common to other simple molecules.
Simple (yet not that simple) fluids
A few years ago we started a collaboration with Dr. John Proctor (University of Salford), who has an interest in supercritical fluids (widely used as drilling agents and as solvents for materials processing). The interest was sparked by the discovery in 2013 of a crossover in the liquid and supercritical fluid region of phase diagrams from a non-rigid to a rigid fluid. This crossover is called a ‘Frenkel line’, and it is primarily visible in changes in dynamical properties of the fluid as it crosses the line.
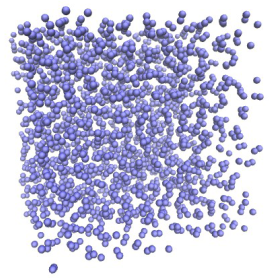
Our neutron scattering measurements on nitrogen and krypton have shown the coordination number of molecules/atoms to be a clear and easily identifiable structural marker for the Frenkel line. The coordination number increases linearly with pressure/density below the line, and shows a tendency to flatten and tend asymptotically to ~12 after crossing the line.
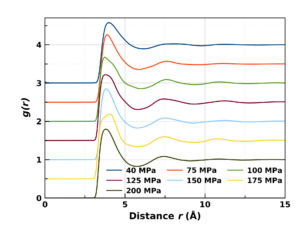
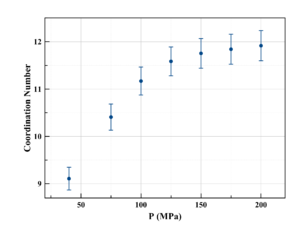
Our studies allowed us to formulate an analytic formula for locating the Frenkel line on phase diagrams (colloquially referred to as the Pruteanu-Ackland criterion), and showed it to be universally applicable to all fluids.
We are now exploring further systems in the hope of locating exactly the origin of the Frenkel line and what phase lines (boiling/melting curve) it has contact with, as well as determining if there is any effect of crossing the Frenkel line on the free-rotor behaviour of diatomic molecules.
In collaboration with Dr. Leo Lue (University of Strathclyde), we have managed to extend the concepts pertaining to the Frenkel line from simple fluids to discontinuous potential systems, such as hard spheres and square well potentials, representative of colloids and colloid-polymer mixtures. This also allowed us to extend the previously formulated analytic criterion to other physical observables such as the diffusion coefficient and velocity auto-correlation function.
Our current efforts are focused on expanding the exploration of the Frenkel line to different classes of discontinuous potential systems.
Classical Interatomic Potentials for Extreme Conditions
We are involved in efforts to produce/refit classical atomic forcefields to become transferable and applicable to high pressure conditions, in the hope of them serving as a cheap screening tool for exotic behaviour.
In collaboration with Prof. Graeme Ackland and Prof. Davide Marenduzzo we are looking at adapting already-existing established forcefields (such as AMOEBA) for quantitative agreement with experimental observables in high pressure fluid mixtures.
Also in collaboration with Prof. Ackland we are using machine learning to train from scratch new potentials. The first iteration of this kind of potential for molecular nitrogen produced quantitative agreement with our neutron measurements on the structure of the supercritical fluid and the known equation of state for nitrogen at those conditions.
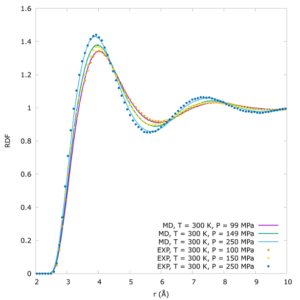
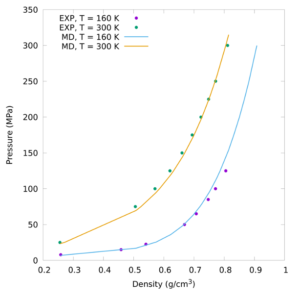